Seizing the Teachable Moment from
the BRF Real Time Data
This webpage is intended primarily
for educators who are planning a class field trip to Black Rock Forest and wish
to make use of the environmental sensor data during their visit.
Introduction for Newcomers to Black
Rock Forest:
The Black Rock Forest (BRF) is a 3750
acre (1500ha) preserve dedicated to scientific research, education, and conservation,
located on the west bank of the Hudson River, 50 miles (80km) north of New York
City. BRF is home to numerous ponds, ridges, wetlands, and great biological diversity.
A consortium
of schools, colleges, and research institutions operates Black Rock Forest, using
it extensively for research on topics
ranging from tree-rings to glacial geology, and for
student field trips for ages ranging from kindergarten through graduate school.
The forest has been instrumented with environmental sensors, which continuously
measure and record properties of the air, soil and water. A suite of student activities
based on data from the sensors have been developed to help students extend their
experience of the forest beyond the few hours of a typical field trip.
The Real Time Data:
"Real Time" data are observational data
that are made available to users immediately after the actual event or condition
being observed has occurred. At Black Rock Forest, our data are actually
"near-real-time" data, because the data are only transmitted from the environmental
sensors to Forest Headquarters once per hour. This data transmission rate was
chosen to conserve power. The transmitters at the sensors are powered by batteries
which are recharged from solar panels. Continuous transmission of data would take
more power than could be supplied by this system.
BRF [near] real time data can be displayed on a computer at the Forest Headquarters.
There it is used by Forest staff to monitor the functioning of the remote
sensor stations, and by scientists to monitor changing conditions in the forest
that may call for an action in an observation campaign or a modification to an
experimental design.
The real time data are also available to educators and school groups visiting
the Forest. These data are most pertinent to classes which are studying, or have
studied, weather, the water cycle, and/or habitats. If you wish to
have access to real-time data during your class field trip, please indicate this
to the Forest staff in advance of your trip.
What can students learn by looking
at real time data?
Probably the most important insight
you can convey from the real time data is that environmental factors vary across
both time and space. At some level students probably know this, but
because they themselves experience only one time and one place at any given moment,
and because their knowledge and awareness of reality may still be quite egocentric,
they may not really KNOW it very deeply. Furthermore, they may not
realize that it is possible to measure and record and methodically examine how
the environment varies from place to place and time to time.
Understanding how air, water and soil vary across time and space is important
for understanding both the causes and the consequences of environmental variability. Thinking about causes leads to questions like: why is it that air
temperature goes up and down on a 24 hour cycle? why is it that one
site consistently has lower relative humidity than the other? Thinking about consequences
leads to questions like: which of these places would be a better habitat
for a particular animal of interest to me?
Teaching with the BRF Real Time
Data:
Because real-time data are different
every day, you cannot prepare in advance by pre-analyzing the data as you could
for an activity built around archived data. You can to turn this to your advantage
by allowing your students watch you think aloud as you observe and describe the
salient properties of the data display. Involve your students in generating hypotheses
about what might cause the data to look the way they do. Emphasize that this data
is brand new; "we are the first people ever to describe and interpret this exact
data set."
Although you cannot prepare by interpreting the data in advance, you can think
in advance about which data you would like your students to look at. Air, soil
and solar radiation measurements are made at two sites: Open Lowland and Ridgetop.
Streamflow is measured in Cascade Brook. It may help to refer to the map
of sensor locations as you plan. The readily-available data types are:
- air temperature
- soil temperature (10cm and 100cm
subsurface)
- relative humidity
- vapor pressure
- atmospheric pressure
- total solar radiation
- photosynthetically-active solar
radiation
- wind speed and direction
- rainfall
- streamflow
Here are some things you may wish to
do with your students during your visit before viewing the real-time data:
- Visit the Open Lowlands
environmental sensor station (behind the old headquarters), and figure
out where all the different sensors are.
- Use hand held instruments to measure
some of the atmospheric parameters you will be observing in the sensor data. Air temperature, atmospheric pressure, and relative humidity are good
candidates.
- Complete the "Sensors/Senses"
activity, which brings out the similarities and differences between electromechanical
sensors and the human body's own senses.
As you and your students first
begin to look at the data, here are some general questions that are pertinent
to most of the BRF real time environmental data displays:
- What is shown along the horizontal
axis? (typically the answer is "time")
- How much time is shown on the
graph? (typically the answer is "two days")
- Where is "now," the current time,
on the graph? (typically the answer is: "at the right
hand edge of the graph")
- Does the graph agree with what
we measured using the handheld instrument? If not, what might be the cause
of the difference? (Example answers: we made our measurement
an hour ago; we made our measurement here at Forest Headquarters and the sensors
are at Ridgetop and it is windier up there.)
- What does the vertical axis show?
(answer varies)
- What are the units on the vertical
axis? (important, but not always obvious. Some two-line
graphs have different units on the left and right axes.)
- Why does the line go down and
up and down and up (or up and down and up and down)? (many
of the environmental measures have a day-night cycle, and that two-peaked
shape represents two days and two nights.) (Note that this is a question about
causes of temporal variation.)
- What would we have felt (or seen)
if we had been here then? [Point to a spot on the graph
with characteristics different from the present.] (Note that this is a question
about consequences of temporal variation.)
Examples:
Air Temperature at Open Lowland
and Ridgetop:
Following are some examples of real-time
data displays, with notes on the kinds of questions that teachers can ask and
the kinds of observations and interpretations that students can make. These
four examples are related around the theme of diurnal variation in air temperature.
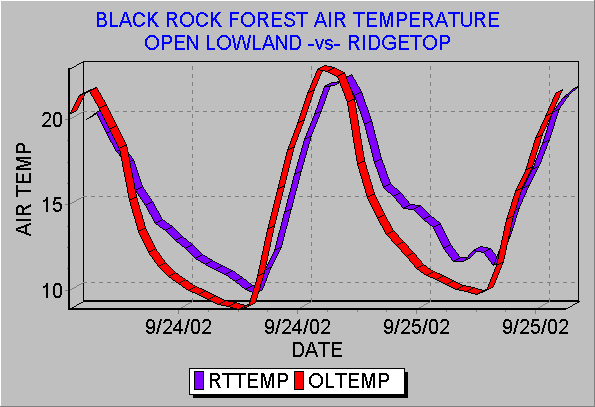
- Why do the curves go up and
down? (The high parts of the curve are daytime warm
temperatures and the low parts of the curve are nighttime cold temperatures.
During the day, the sun is shining on our side of the Earth, providing
energy, which warms the air (indirectly). During the night, our side of
the planet is turned away from the Sun, there is no energy coming into
the system, and heat escapes from the atmosphere into space.)
- Why are there two curves,
red and blue curves? (The red curve is data from
the Open Lowland site and blue curve is data from the Ridgetop site.)
- What was the warmest temperature
during this time interval and where did it occur? (approximately
23 degrees Celsius, at the Open Lowland site, on September 24.)
What was the coldest temperature during this time interval and where did
it occur? (approximately 9 degrees Celsius, at the
Open Lowland site, on September 24.)
- What differences can you see
between the temperature pattern at the Open Lowland site and the Ridgetop
site? (Open Lowland got hotter in the daytime and
cooler at night than did Ridgetop. Open Lowland heated up faster in the
morning and cooled down faster in the evening than
did Ridgetop.)
- Can you suggest any explanation
[hypothesis] for why Open Lowland got hotter in the daytime and colder
at night than did Ridgetop? (There is no single
right answer that is obtainable from the data at hand. Encourage hypotheses,
and then ask "how could we test this hypothesis?)
Air Temperature and Solar Radiation:
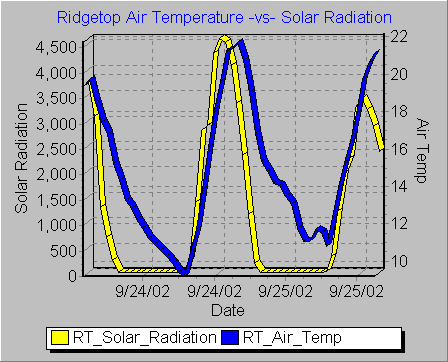
- What are the two curves?
(Blue is the air temperature, and yellow is the
amount of solar radiation. Both are at the same site, Ridgetop.)
- What's going on in this
flat part of the solar radiation curve? (The flat
part of the curve represents zero in coming solar radiation, in other
words it is dark out, in other words it is night time.)
- When does the peak in solar
radiation occur? (At noon, at the moment when the sun is highest in the sky. If
you have previously taught about how sun angle influences solar energy
received at the Earth's surface, you could now ask why does the angle
of the sun matter?)
- If we were to look at many
days worth of data, do you think the peak of solar radiation would always
be at noon? (Not necessarily; some days would
be cloudy and noon and sunny in the morning or afternoon. In that case,
the peak of solar energy could be at an uncloudy moment in the morning
or afternoon, even though the sun wasn't highest in the sky at that
moment.)
- Where is sunrise on the
graph? (at the point where the solar radiation
curve stops being flat and starts rising steeply) Where
is sunset?
- When is the coldest time
in each 24 hour day? (Just before the dawn.)
- Why is the coldest time
just before dawn? (All through the night the Earth
loses heat into space, and the temperature drops continuously. When
the sun rises, it begins to heat the rock and soil, the rock and soil
re-radiate heat into the overlying air, and the temperature begins to
rise.)
- Why doesn't the air temperature
plunge to zero when the solar energy input goes to zero?
(The molecules of the air and soil and rock still contain heat energy
in the form of vibrating molecules, even though no new energy is coming
into the system at night. Also, the data shown are on the Celsius scale.
Zero degrees Celsius is the point at which water freezes, an arbitrary
convenient choice of where to set the zero of the temperature scale.
Zero degrees Celsius does not mean zero heat energy in the same way
that $0 in your bank balance means zero dollars in your account.)
- When is the hottest time
in each 24 hour day? (An hour or two after noon,
an hour or two after the peak in solar radiation.)
- Why is the hottest time
of day after noon rather than exactly at noon? (Sunlight
does not heat the air directly, at least not very much. Instead, soil
and rock absorb the light from the sun and re-radiate it as heat energy,
infrared waves. This re-radiated energy is what does the work of heating
up the overlying air. This process of re-radiating takes time and extends
over time, and introduces a lag between the peak in arrival of solar
energy and the peak in air temperature.)
Air Temperature and Soil Temperature:
- What are the two curves?
(One is air temperature, the other is soil
temperature. Both are at the Ridgetop site.)
- What differences can
you see between the air temperature pattern and the soil temperature
pattern? (The air temperature changes drastically
from day to night, by about 12 degrees Celsius. The soil temperature
also changes on a 24 hour cycle, but only by about two degrees.
The daily high and lows of temperature in the soil occur after the
corresponding highs and lows in the air temperature.)
- Can you suggest a hypothesis
to explain the differences between the air temperature and soil
temperature patterns? (During the day, the
sun warms the top layer of the soil. Heat conducts from the top
layer of soil into the soil beneath. Soil isn't a good heat conductor,
so this process of heat conduction through soil is slow and inefficient.
Also, it takes a lot of heat to warm up a given volume of soil,
especially wet soil. Thus the soil at 10cm beneath the surface doesn't
get very warm. At night, heat is lost from the soil into the cool
air, and the soil temperature drops. But again, this process is
slow, and so the soil doesn't cool down very much during the course
of a single night. The top layer of soil insulates the deeper layers
from the extremes of both heat and cold in the air above.)
- Does this data suggest
any reason why an animal might want to live in a hole in the ground.
(A burrowing animal can escape from extreme
heat during the day and from extreme cold during the night.)
Relative Humidity at Open
Lowland and Ridgetop:
- What are the two curves? (Red
is relative humidity at Open Lowland, and blue is relative humidity at Ridgetop.)
- What is relative humidity? (The
ratio between how much water vapor is actually in the air and how much water
vapor would be in the air at saturation.)
- What is the highest relative humidity
that occurred in this data set? (100%, or just a shade
under 100%)
- Where and when did that maximum
humidity, 100%, occur? (At the Open Lowland site, in
the early morning hours just before dawn.)
- If you had been at the Open Lowland
site in those early morning hours just before dawn, what would you have observed?
(It would have been dark and cold. Everything would
have been coated with dew. Dew is formed by water condensing out of the atmosphere
when the air is water saturated, in other words when the relative humidity
is 100%.)
- What is different about the pattern
of relative humidity at Ridgetop from that at Open Lowland? (The
Ridgetop site never reached saturation (100% relative humidity) during the
time interval represented by this data set.)
- Can you suggest some reasons why
the air at the Ridgetop site did not reach water vapor saturation during the
time shown by this data set? (Encourage plausible reasoning
and generation of multiple working hypotheses. Possibility #1: The exposed
Ridgetop site is windier, and the wind continuously brought in fresh undersaturated
air. Possibility #2: the stream near the Open Lowland site contributed moisture
to the air. Possibility #3: the Ridgetop site remained warmer through the
night (see air temperature record), and thus did not reach the "dewpoint,"
the temperature at which liquid water begins to condense out of air of a given
vapor pressure.)
Created by Kim Kastens, Lamont-Doherty
Earth Observatory (kastens@ldeo.columbia.edu).
May be freely used for educational purposes provided appropriate credit is given.
Last
modified
November 5, 2003
Return
to the Black Rock Forest Student Investigations Home Page