U.S./Chinese Collaborative Study: Investigation of Bottom Water Formation in Prydz Bay, Antarctica
The field program will be carried out on board of R/V Xuelong during her annual trip to service Chinese Antarctic Stations.
Program Description
Supported by National Science Foundation of United States and Chinese Arctic
and Antarctic Administration, scientists from Lamont-Doherty Earth
Observatory of Columbia University (Lamont), First Institute of Oceanography (FIO) and
Polar Research Institute of China (PRIC)
will collaboratively conduct three seasons of physical oceanography surveys in Prydz Bay, Antarctica to investigate the
bottom water formation processes.
Sponsors
National Science Foundation
United States of America
Chinese Arctic and Antarctic Administraiton
State Ocean Administration
People's Republic of China
Principal Investigators
Xiaojun Yuan
Lamont Associate Research Professor
Lamont-Doherty Earth Observatory of Columbia University
The United States
Libao Gao
First Institute of Oceanography
State Ocean Administration
People's Republic of China
Bingrui Li
Director of Physical Oceanography division
Polar Research Institute Of China
State Ocean Administration
People's Republic of China
Field Work
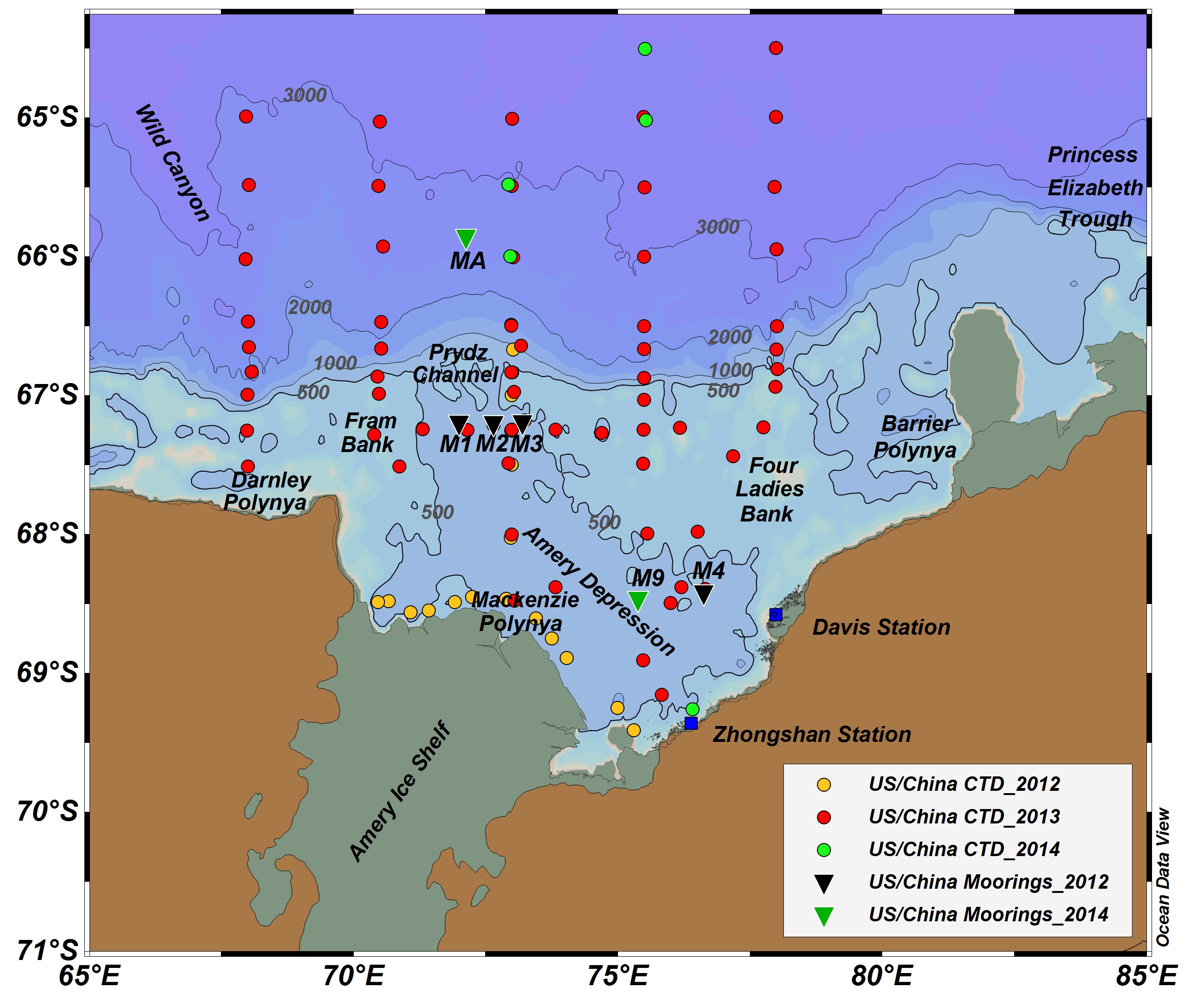 Figure 1. Summary of hydrographic surveys by US/Chinese
collaborative research in the Prydz Bay region. Mooring M1 to M4 were originally deployed in 2/2012. M1 and M3 mooring were recovered and redeployed in 2/2013. M9 and MA were deployed in 3/2014.
Lamont Mooring M1
|
Preliminary Results
To investigate the AABW production in the Prydz Bay region, the program has conducted three seasons of fieldwork in 2012, 2013 and 2014 during XueLong's annual resupply trip (figure 1). Two CTD sections (yellow dots) were occupied and four moorings (M1, M2, M3, and M4) were deployed in 2012. In 2013, the team conducted 6 CTD sections (red dots) and redeployed M1 and M3. The fieldwork during February 2014 was only partially completed due to heavy sea ice, which prevented mooring recovery. XueLong managed to deploy two more moorings (M9 and MA) and conducted limited CTD (green dots). The data collected in 2012 and 2013 have been fully processed and preliminary results are presented here. The CTD section along 73°E was sampled in both 2012 and 2013, which captured mCDW entering the shelf region with similar behavior in both years. The mCDW with a temperature of -0.6°C and a salinity of 34.55 at the shelf break encounters the colder and fresher shelf upon its entering and flows upward on its southward intruding path, suggesting that it is less dense than the shelf water in summer.
However, its salinity is higher than the shelf water, contributing to the formation of dense shelf water in winter. Its contribution depends on the total volume of mCDW import through a year. Figure 2 reveals the spatial extent of mCDW on the shelf break, which mainly occurs between 71°E and 77°E with a depth of center intrusion at 300m. As recorded by the M1 mooring sensors, the depth of the intrusion center varies between 272m and 412m throughout the year. Based on daily mooring CTD data at 272 m (figure 3), we use T>-1.7°C and S>34.5 as the criteria to define a mCDW intruding event. Such intrusion occurred in 48 days out of 378 days of sampling. Most intrusion events are short-lived and eighty-one percent of them last only 1-4 days. In addition, the intruding events peak when sea ice is very low (January through April) and do not occur from May to August when the area is close to be completely covered by sea ice. To investigate the relationship between surface winds and mCDW intrusions, we calculated the wind stress curl using ERA-Interim reanalysis data. The mean curl of wind stress in a 1x1 degree area around M1 is positive 95% of days during the sampling period but mCDW events only occurred in 11% of the days. Therefore, local Ekman suction is not a deterministic factor of inducing the intrusions. (Gao et al., in preparation). The mechanisms that lead to mCWD intrusions remain unknown at this location.
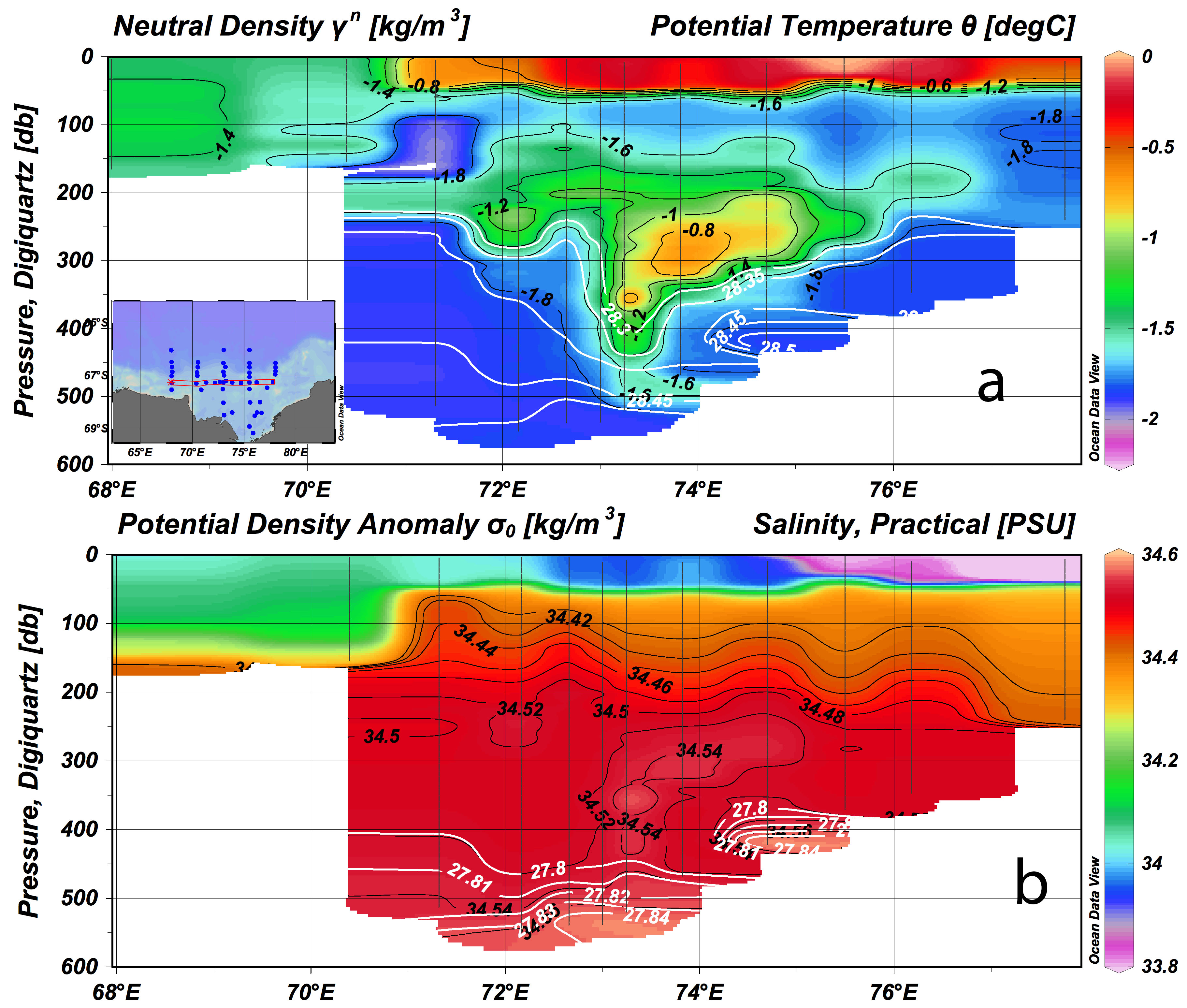 Figure 2 Potential temperature (a) and salinity (b) along shelf break occupied in 2/2013. Neutral density and potential density are superimposed in (a) and (b), respectively.
The time series at this location can be classified into four periods based on local water mass characteristics (figure 3). Period I (2/8/12-4/11/12) is from summer to early fall and characterized by a moderate number of mCDW intrusions and mean temperature above the freezing point. It is followed by period II (4/11/12-7/31/12) with temperature at the freezing point (implication of ice formation). ISW passes through this location from May to August during period II, which overcomes the salt injection from ice formation and reduces the mean salinity by 0.01 from period I, resulting in slight reduction in density. Between period II and III, there is an intensive ice formation event, increasing salinity from 34.48 to 34.57 and density from 27.76 to 27.83 kg/m 3 in two days.
The salinity jump is equivalent to 1.5m of sea ice formation, assuming that shelf water is mixed from top to bottom in August and the mean shelf water salinity is 34.50. This extreme ice formation event, together with moderate number of mCDW intrusion events and ice formation during August and September, elevate the mean salinity by 0.02 and the mean density by 0.015 kg/m 3 in period III. Period IV (1/1/13-2/20/13) is characterized by frequent mCDW intrusions. Without any ice formation, these intrusions effectively bring the mean salinity 0.01 higher than that in period III. Even that the mean density does not increase due to warmer temperature, the higher salinity in the water column contributes to the production of dense shelf water in following winter. Clearly both ice formation and mCDW can competently change salinity near the shelf break, their relative roles in producing dense shelf water still need to be quantified.
Dense shelf water exists on the Prydz Bay shelf region even in austral summer. The lower water column (approximately 200m thick) has potential density higher than 27.80 kg/m3 with maximum density of 27.84 kg/m3 near the shelf break (figure 2). If it were exported off the shelf, it would be dense enough to descend along the slope. Figure 4 shows the spatial distribution of the dense shelf water in February 2013.
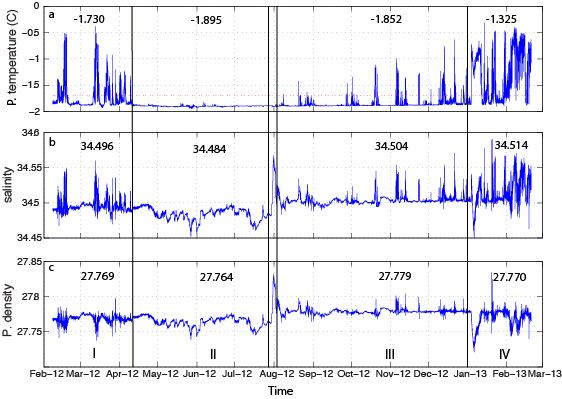 Figure 3. Potential temperature (a), salinity (b) and potential density (c) at 272m on M1 from 2/12/2012 to 2/12/2013 with a sampling rate of 15-minute. The vertical lines separate measurements into 4 periods according to their characteristics. The mean values of temperature, salinity and density in each period are marked in each panel.
It is mainly concentrated in the Amery Depression, where the topography is 800-900m deep. Temperature is close to the surface freezing point, while salinity reaches 34.6 in the depression, consistent with winter elephant seal data (Ohshima et al., 2013),. Oxygen near the bottom is above 6.6 ml/l in most of shelf region, suggesting that the bottom layer is well ventilated in winter. The bottom density is mostly higher than 27.80 with a maximum of 27.86 kg/m 3 along the west limb of the depression. Although the bottom salinity in the Prydz Bay shelf region is not as high as that in the Cape Darnley polynya in winter, the density of shelf water is higher than the density on the slope and would sink down the slope upon being exported.
The circulation in the bay also favors export at Prydz Channel. M1 current meter at 347m (figure 1) recorded an annual mean speed of 7+/- 4 cm/s and mean direction of 10+/- 21 degree from north. The current does not have a clear seasonal cycle but the maximum speed can reach 31cm/s. The volume of dense shelf water is determined by amounts of mCDW intrusion and sea ice production. Estimates of salt injection from ice formation and salt fluxes delivered by mCDW are currently being constructed for the period of 2/2012 to 2/2013 using data from M1 and M3 moorings, surface winds from reanalysis and sea ice observations (Yuan et al., in preparation).
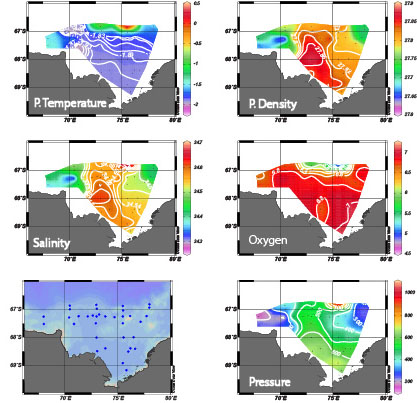 Figure 4. Water properties and pressure near the bottom of water column based on CTD data in February 2013.
However, these sparse in situ observations could not fully depict the season cycle of dense water production and export rates. A high-resolution regional ocean-sea ice coupled model that incorporates observations is needed for understanding the shelf processes and shelf/slope interaction that lead to the dense shelf water formation/export and bottom water formation.
The AABW in the deep basin beyond 2000 m has a distinct spatial pattern in all water properties. Separated by a north-south oriented subsurface ridge extending from Cape Darnley, AABW at the bottom of water column is colder, fresher, denser and richer in oxygen west of the ridge than that east of the ridge. Property differences across the ridge are 0.4°C for θ3, 0.03 for salinity, 0.03 kg/m 3 for neutral density (or σ3), and 0.3ml/l for oxygen, respectively.
These property contrasts indicate that locally formed AABW (Cape Darnley bottom water) is guided by topography and flows northwest. East of the ridge, AABW was formed in remote source areas. Based on the historical LADCP data and bottom properties, we create a schematic flow pattern in the deep ocean basin (figure 5) to illustrate sources of AABW in the region: Weddell Sea AABW (blue arrows) is transported eastward in the northern part of the study area, while AABW formed in the Ross Sea and Adélie Land coast region (red arrows) is transported westward near the slope. AABWs from these two source regions are mixed in a cyclonic gyre circulation north of Prydz Bay. Locally formed Cape Darnley AABW (yellow arrows) flows all the way down to the deep basin beyond 3000m and turns westward (Sun et al. 2014).
Our current results seem to suggest that any dense shelf water exported from Prydz Bay may not be dense enough to descent to the deep basin beyond 2000 m. Instead, it could flow westward on the slope to join the down slope flow west of Cape Darnley. The total AABW production rate in the region is yet to be quantified, particularly in the changing environment of freshening shelf water and warming deep water around Antarctica and in the Southern Ocean.
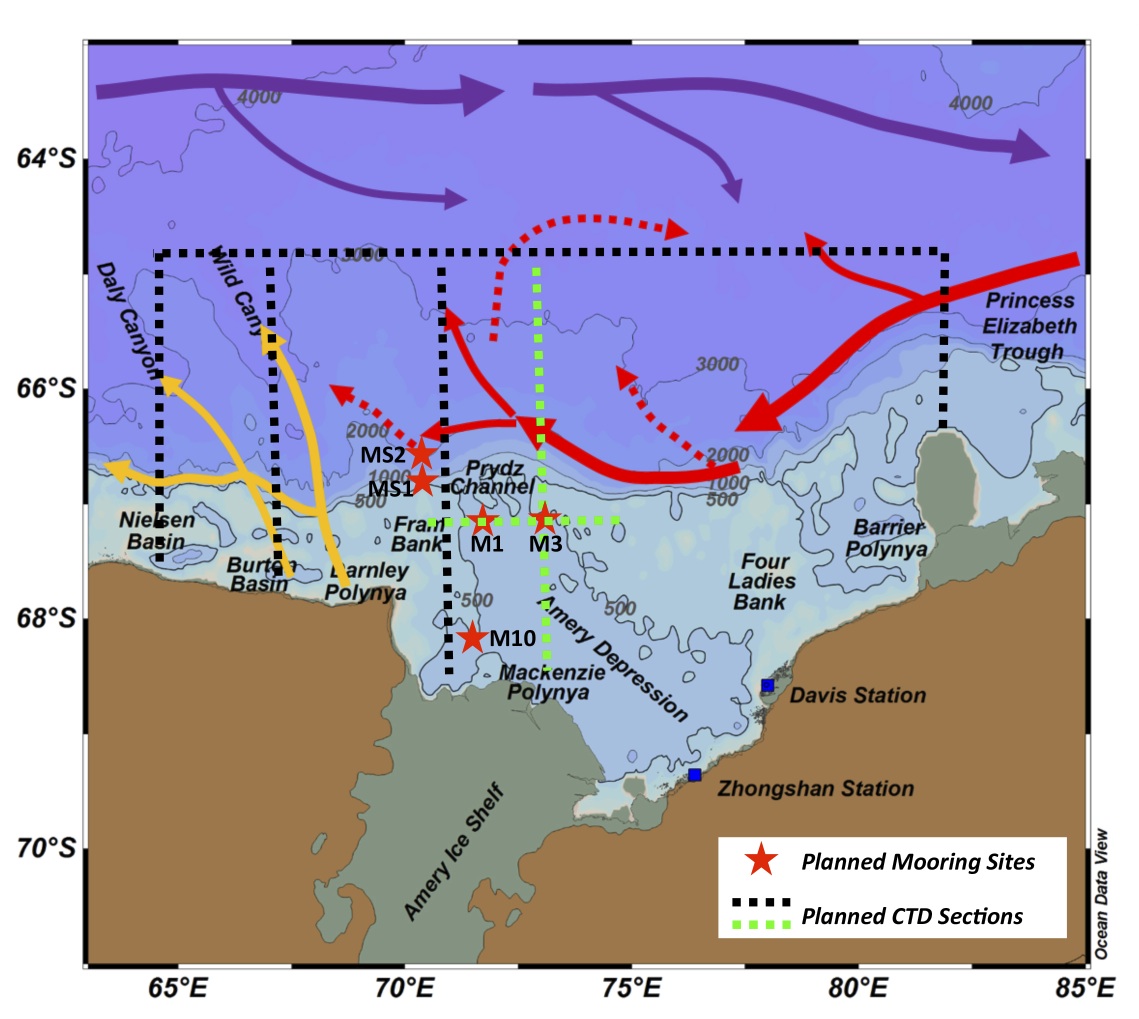 Figure 5 Schematic AABW flow pattern. AABW originated from the Weddell Sea (blue arrows), from Ross Sea/Aledie Land coastal region (red arrows) and from Cape Darnley (yellow arrows) are color-coded. Proposed subsurface moorings (MS1,MS2,M1,M3 and M10) are marked in red stars, while black and green dached lines indicate proposed CTD/LADCP sections.
Data
CTD Data
2012
2013 - in progress
2014 - in progress
Publications/Presentations
Sun, Y., X. Yuan, J. Shi, L. Gao, Changes of Antarctic Bottom Water Properties in the Prydz Bay Region, Poster presentation, The Ocean Science Meeting, Honolulu, 2014.
Gao, L., W. Guy, X. Yuan. Variability of Deep Circumpolar Deep Water inflow onto the Prydz Bay shelf region. In preparation.
Yuan, X. L. Gao, Y. Sun, et al., Spatial and Temporal variability of shelf water in Prydz Bay. In preparation.
|